Determination of the operation mechanism of the proteasome, a macromolecular protein degradation enzyme, and unraveling the relationship between proteasome functional abnormalities and disease (cancer, inflammation, aging, neurodegeneration)
The proteasome is one of the intracellular protein degradation enzymes. The proteasome mainly degrades proteins tagged with an ubiquitin chain by the ubiquitin system; the ubiquitin chain serves as a degradation signal. Taken together, this system is called the ubiquitin proteasome system. The strong point of this degradation mechanism is that proteins that need to be degraded are found at pinpoint precision, and degraded at just the right time. This protein degradation system plays a central role in numerous cellular activities such as the cell cycle, signal transduction, and transcriptional control (Fig. 1).
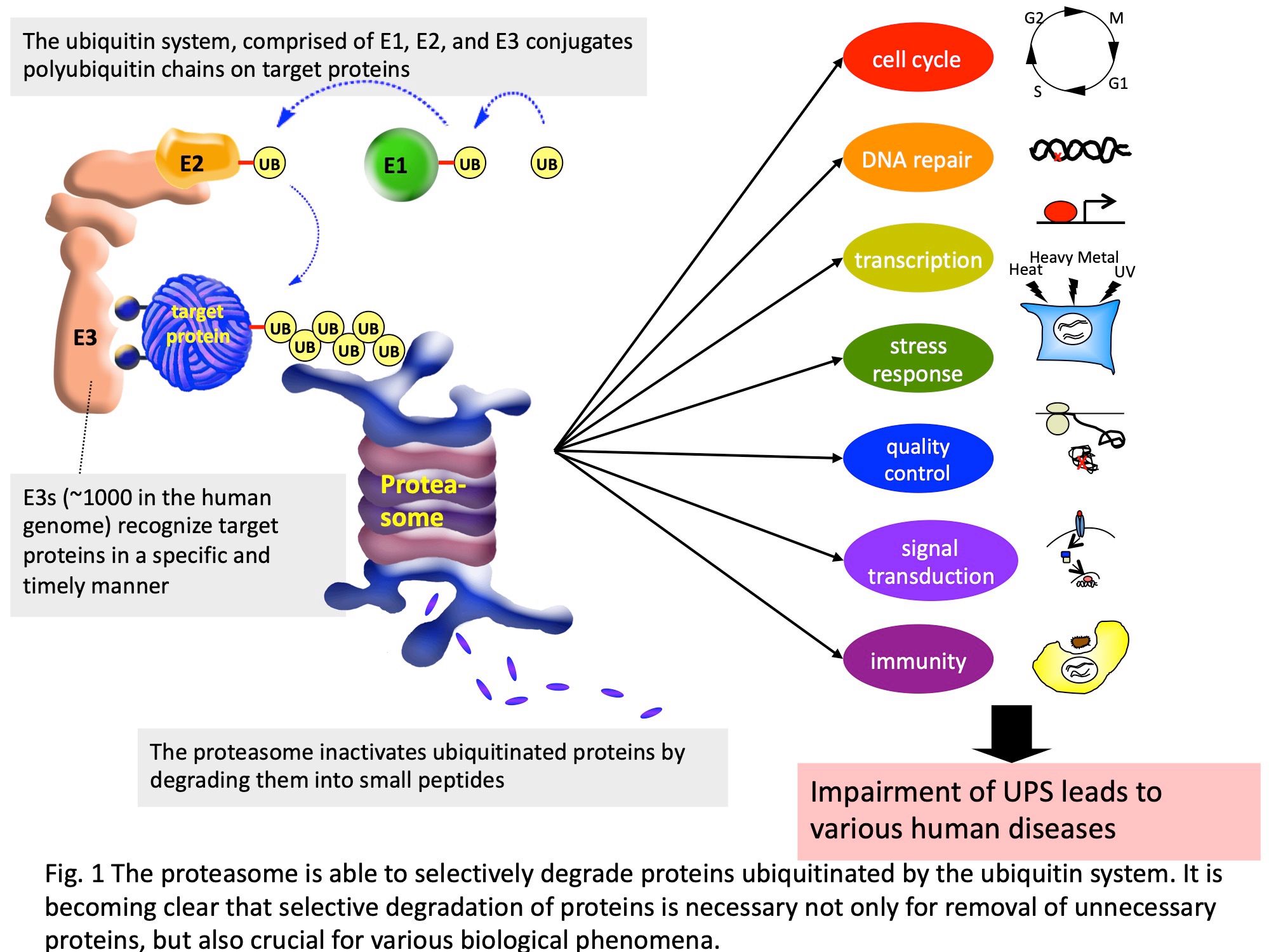
The proteasome is a colossal enzyme made up of a total of 33 different types of subunits, with a total of 66 subunits (Fig. 2). The proteasome is precisely and logically assembled to perform the recognition and capture of ubiquitin chains, removal of ubiquitin chains, unfolding and transport to the active center, and protein degradation all within a single proteasome (Fig. 3). However, how such a complex structure was accurately assembled remained a large mystery. To date, we have found numerous specific chaperones that assist in proteasome assembly, and revealed the detailed mechanism of proteasome assembly.
Furthermore, recently, we have discovered that this assembly pathway plays a pivotal role in regulating the amount of proteasomes according to the state of the cell. From the viewpoint of regulation of the amount of proteasomes, the transcriptional regulation of proteasome subunits is also largely unknown. Loss of control of proteasome amount is linked with the pathology of cancer, neurodegenerative disease, and aging. By continuing to pursue mechanisms of regulation of proteasome expression and assembly, we aim to unravel the mechanism of intracellular proteasome expression regulation and the link between proteasome levels and disease to develop novel strategies for the treatment of the above diseases.
Currently, drug development targeting the proteasome has been most effective in treating cancer. Cancer cells are known to produce abundant proteasomes for their survival, and the proteasome inhibitor bortezomib has proven highly effective against multiple myeloma. By development of “drugs that prevent increase of proteasome levels,” novel treatments for cancer could be developed.
In contrast to cancer, a decrease in proteasome function is related to aging and neurodegeneration. It is known that proteasome function decreases with age, but recent surprising reports have found that in model organisms such as D. melanogaster and C. elegans, when a decline of proteasome function was prevented, lifespan expansion and resistance to neurodegeneration were observed. This suggests that starting with neurodegeneration, a decrease in proteasome function is linked with numerous aging-associated diseases. Thus, development of “drugs that increase proteasome activity” may present novel treatments for symptoms of aging and neurodegeneration.
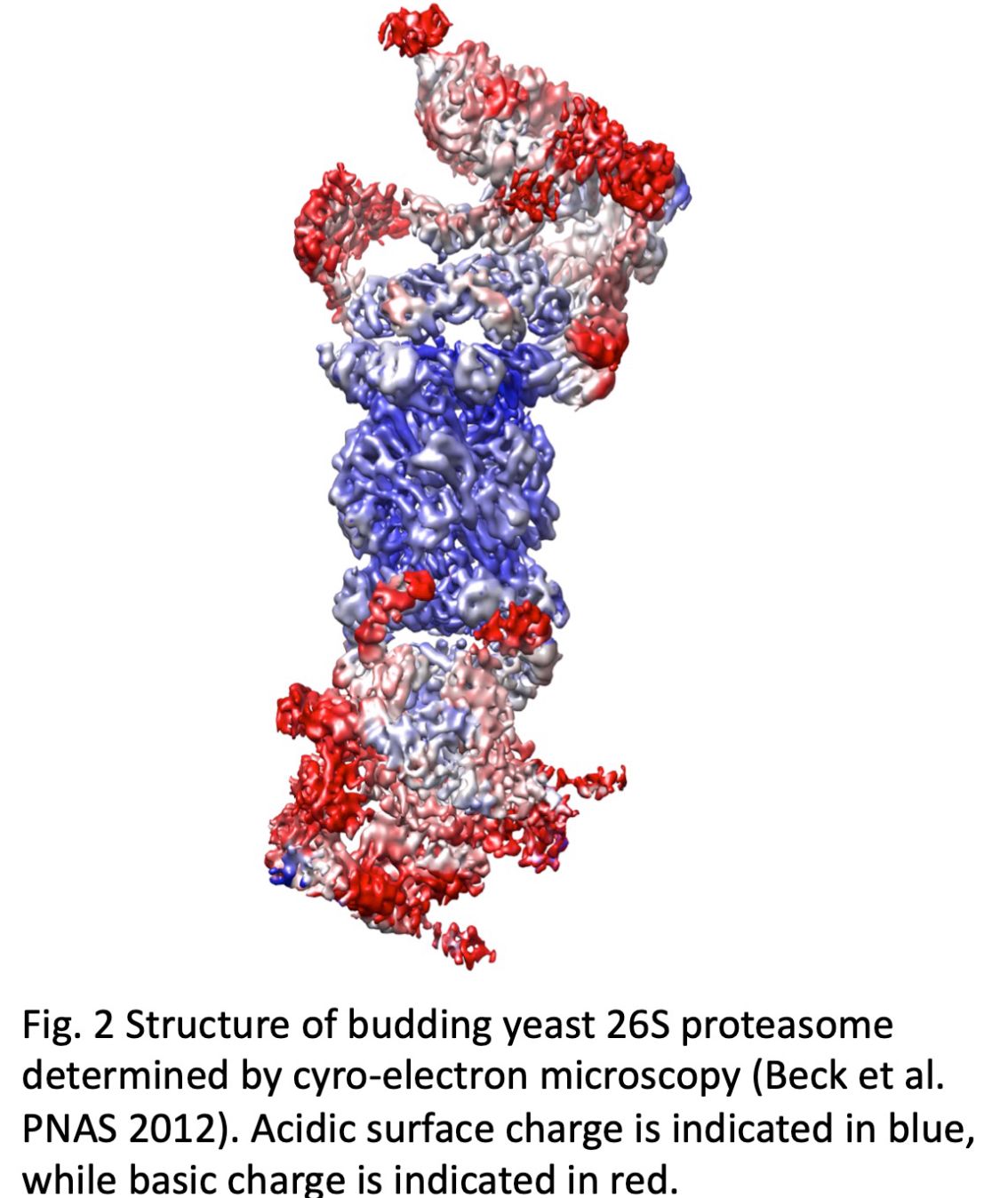
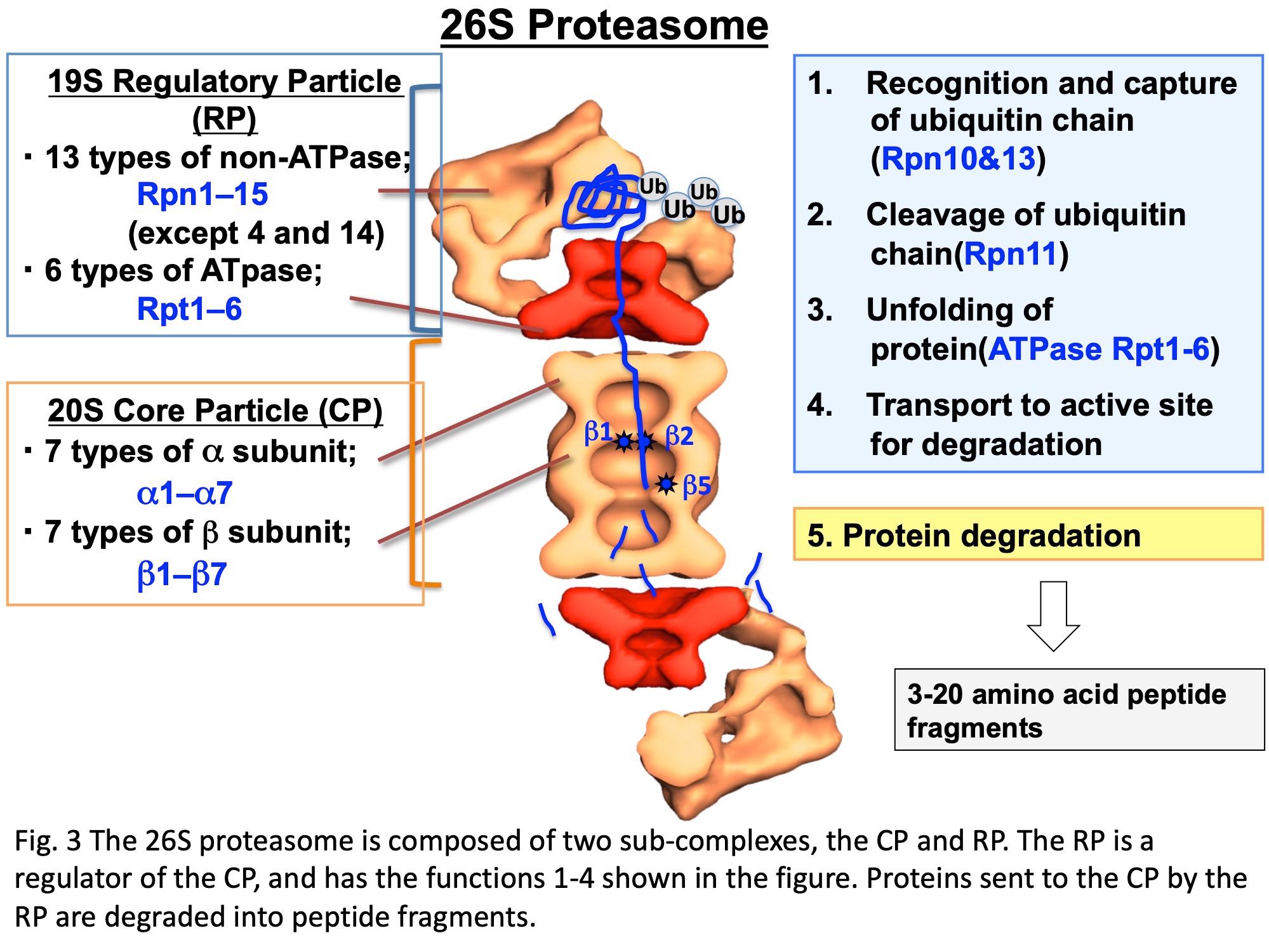
Currently, drug development targeting the proteasome has been most effective in treating cancer. Cancer cells are known to produce abundant proteasomes for their survival, and the proteasome inhibitor bortezomib has proven highly effective against multiple myeloma. By development of “drugs that prevent increase of proteasome levels,” novel treatments for cancer could be developed.
In contrast to cancer, a decrease in proteasome function is related to aging and neurodegeneration. It is known that proteasome function decreases with age, but recent surprising reports have found that in model organisms such as D. melanogaster and C. elegans, when a decline of proteasome function was prevented, lifespan expansion and resistance to neurodegeneration were observed. This suggests that starting with neurodegeneration, a decrease in proteasome function is linked with numerous aging-associated diseases. Thus, development of “drugs that increase proteasome activity” may present novel treatments for symptoms of aging and neurodegeneration.
Elucidation of the selection mechanism of T cells by thymus-specific proteasomes
Acquired immunity is an elegant system that distinguishes between self and non-self, attacking only non-self. T cells are central to this specific response. Our bodies are protected by maintaining a set of T cells (called a repertoire) that can attack a wide range of pathogens entering the body, while at the same time not attacking our own healthy cells.
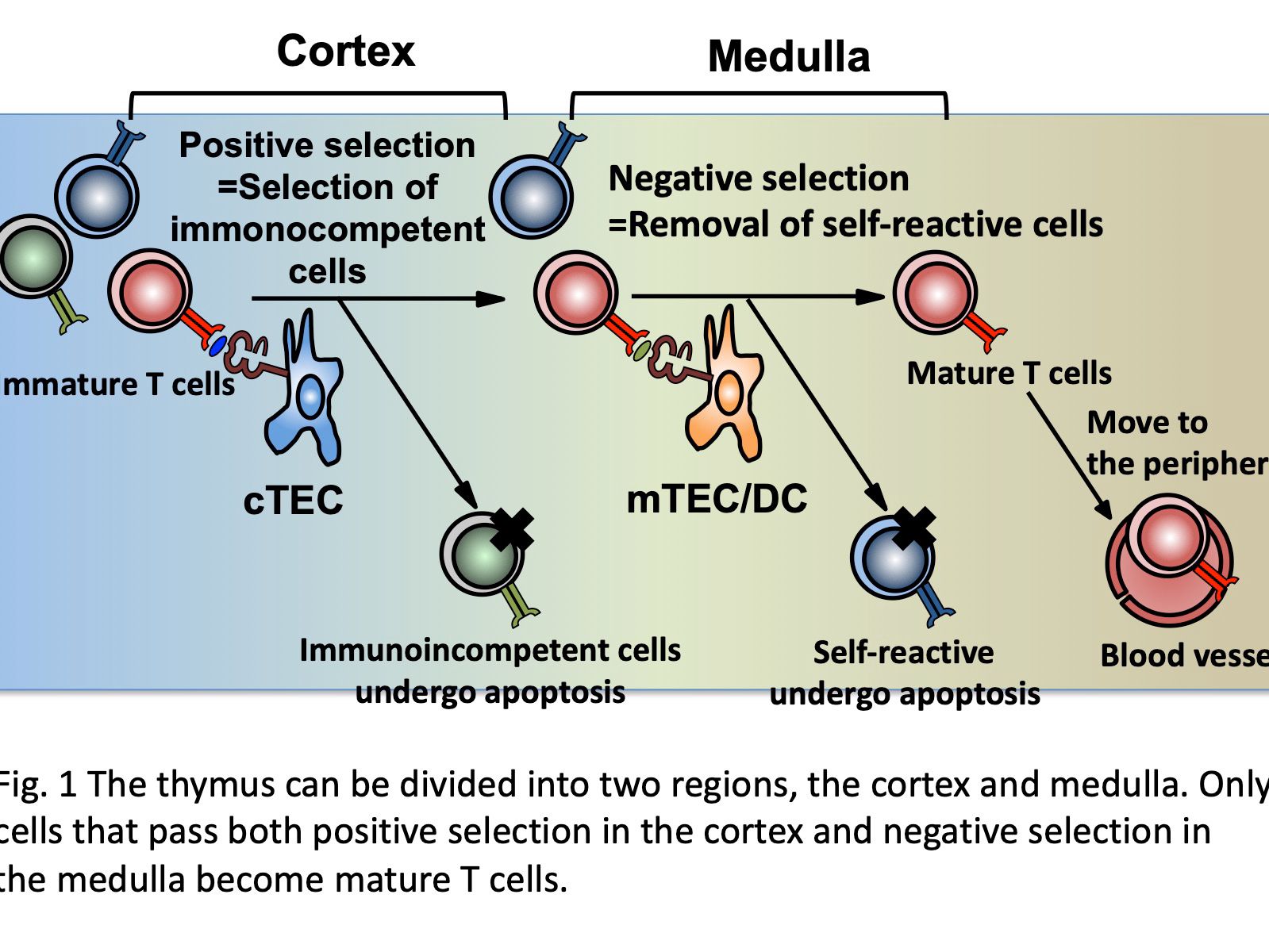
Individual T cells express only one type of T cell receptor (TCR) on the cell surface. Through interacting with the major histocompatibility complex (MHC) on other cells’ surface, this T cell receptor determines whether to eliminate a cell. An indispensible piece of information for this process is a peptide fragment that is bound to a groove in the MHC and presented to the TCR. By presenting this peptide fragment along with the MHC, it can be determined whether non-self agents have affected self-cells.
In order to respond to foreign pathogens that enter our body, TCRs have an enormous range of variety. T cells differentiate and grow in the thymus, an organ located above the heart. First, random gene reconstruction (the same system that creates diversity in antibodies) creates diverse TCRs on the order of 10 to the xx power. From this ensemble, only T cells with a TCR that does not attack self but has the potential to recognize and attack non-self are selected to create a useful T cell repertoire. This process is carried out through “positive selection” and “negative selection.” Only useful T cells are allowed to survive through selection by cortical thymic epithelial cells (cTEC) (=positive selection), followed by removal of cells that react strongly to self-components through selection by medullary thimic epithelial cells (mTEC) and dendritic cells (DC) (=negative selection).
This positive selection and negative selection are performed by cTEC and mTEC based on the interaction between antigen presentation of self-antigen peptides and the MHC complex with individual T cell TCRs. Cells selected by peptide/MHC class I complex differentiates into CD8+ T cells (killer T cells), while cells selected by peptide/MHC class II complex differentiates into CD4+ T cells (helper T cells). Understanding of the molecular basis for negative selection has progressed with discoveries such as that of the transcription factor AIRE (autoimmune regulator), which makes mTEC express organ-specific antigens, suggesting that even while specific to the thymus, mTEC functioned as a “department store of self-components” and made negative selection effective. On the other hand, much remained unknown about positive selection, and many questioned whether there was a specific pathway for positive selection, or for the necessity of the positive selection process altogether.
Recently, we have discovered a cTEC specific proteasome catalytic subunit β5t, and a “thymoproteasome” which contains β5t as a catalytic subunit. In β5t knockout mice, positive selection of CD8+ T cells was severely impaired with an 80% decrease in number of CD8+ cells, and the remaining limited T cell repertoire was an ineffective repertoire unable to mount a proper immune response (Science 2007, Immunity 2010).
In order to respond to foreign pathogens that enter our body, TCRs have an enormous range of variety. T cells differentiate and grow in the thymus, an organ located above the heart. First, random gene reconstruction (the same system that creates diversity in antibodies) creates diverse TCRs on the order of 10 to the xx power. From this ensemble, only T cells with a TCR that does not attack self but has the potential to recognize and attack non-self are selected to create a useful T cell repertoire. This process is carried out through “positive selection” and “negative selection.” Only useful T cells are allowed to survive through selection by cortical thymic epithelial cells (cTEC) (=positive selection), followed by removal of cells that react strongly to self-components through selection by medullary thimic epithelial cells (mTEC) and dendritic cells (DC) (=negative selection).
This positive selection and negative selection are performed by cTEC and mTEC based on the interaction between antigen presentation of self-antigen peptides and the MHC complex with individual T cell TCRs. Cells selected by peptide/MHC class I complex differentiates into CD8+ T cells (killer T cells), while cells selected by peptide/MHC class II complex differentiates into CD4+ T cells (helper T cells). Understanding of the molecular basis for negative selection has progressed with discoveries such as that of the transcription factor AIRE (autoimmune regulator), which makes mTEC express organ-specific antigens, suggesting that even while specific to the thymus, mTEC functioned as a “department store of self-components” and made negative selection effective. On the other hand, much remained unknown about positive selection, and many questioned whether there was a specific pathway for positive selection, or for the necessity of the positive selection process altogether.
Recently, we have discovered a cTEC specific proteasome catalytic subunit β5t, and a “thymoproteasome” which contains β5t as a catalytic subunit. In β5t knockout mice, positive selection of CD8+ T cells was severely impaired with an 80% decrease in number of CD8+ cells, and the remaining limited T cell repertoire was an ineffective repertoire unable to mount a proper immune response (Science 2007, Immunity 2010).
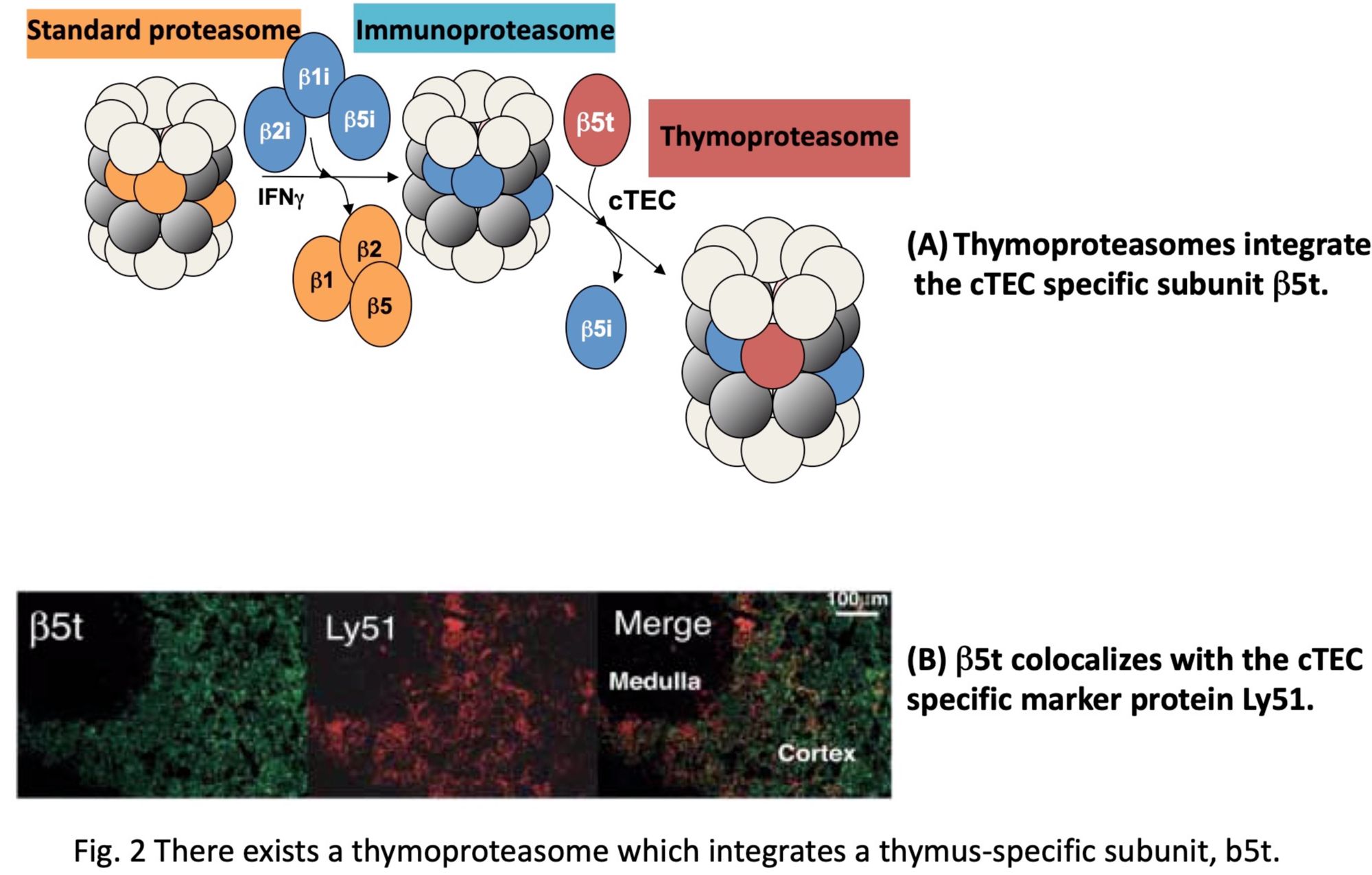
The proteasome is the enzyme responsible for producing MHC class I binding peptides. The proteasome degrades proteins and excretes peptides 3 to 20 amino acids in length. Of these peptide fragments, a subset with the appropriate length and sequence features bind to the groove of MHC class I. Based on the fact that thymoproteasomes have a different peptidase activity from previously discovered constitutive and immunoproteasomes and on analysis results of T cells of various TCR transgenic mice crossed with β5t knockout mice, we have reported the possibility that the thymoproteasome produces special peptides necessary for positive selection. Taken together, we predict that thymoproteasomes produce MHC class I binding peptides in cTEC distinct from other cells, and produce a unique interaction between peptide/MHC class I complex and TCR.
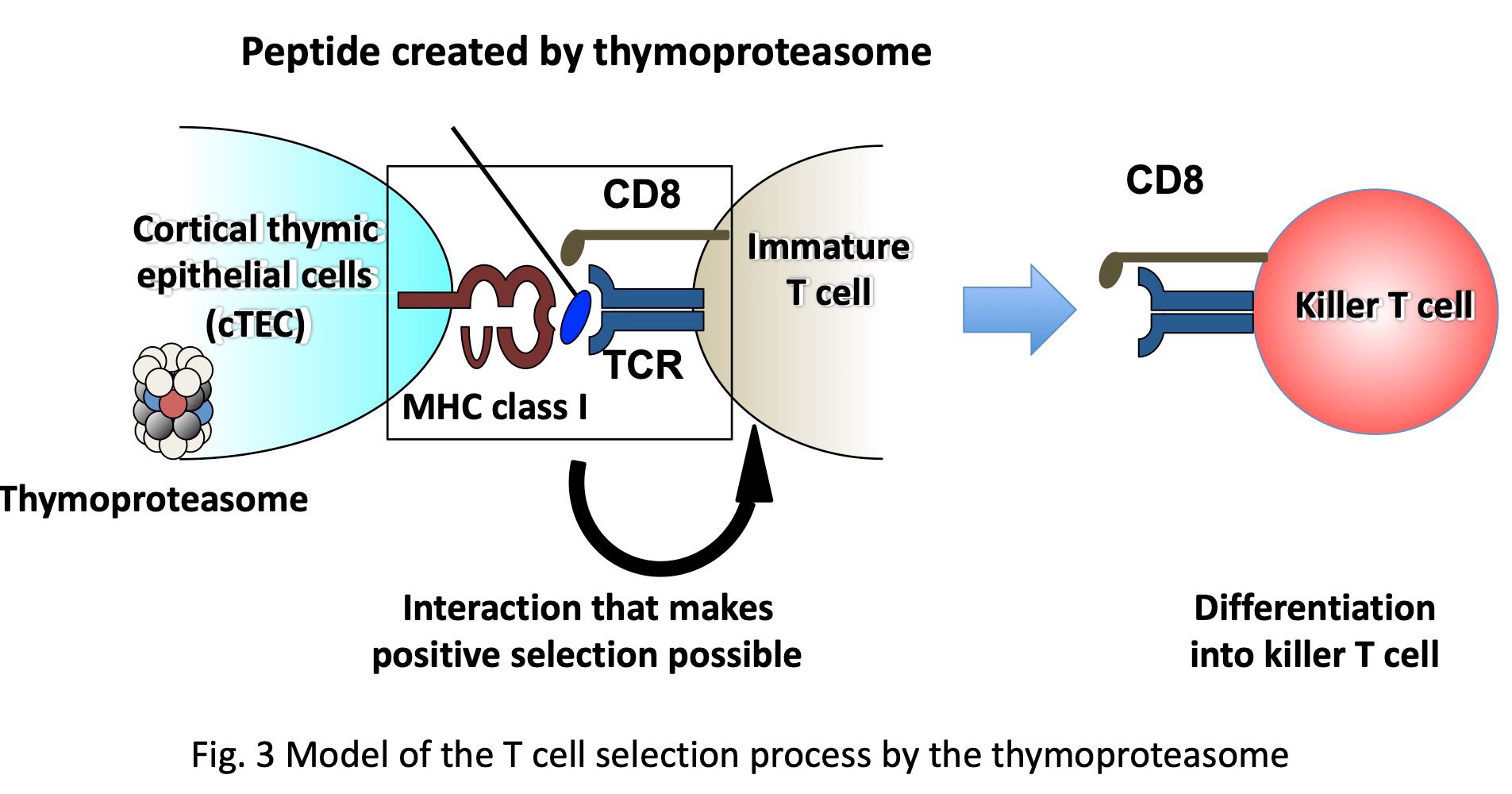
It is likely that the peptides produced by thymoproteasomes provide the proper interaction for “positive selection” to immature T cells and promote their differentiation into CD8+ T cells.
We aim to elucidate the molecular mechanism of the positive selection process through detailed analysis of peptides presented by MHC class I on cTEC.
Unraveling protein quality control mechanisms by the ubiquitin system
Apart from water, proteins make up the second most abundant component of our bodies (60 – 70% water, approximately 20% protein). Indeed, proteins perform a wide range of biological functions, such as through enzymes or shaping of our bodies. Proteins are synthesized at ribosomes based on the information in mRNA by connecting 20 types of amino acids into a string-like structure. A protein only becomes functional upon proper folding of this “string” into a proper structure.
It is estimated that a significant amount of newly synthesized proteins (it has been reported to be between 10 – 30%) fail at folding into their native structure and are thus defective. In addition, even properly folded proteins are constantly exposed to structural abnormality promoting stresses (e.g. heat, UV radiation, low oxygen, reactive oxygen species). However, such misfolded proteins are almost undetectable in cells. This is because of a “protein quality control system.” The ubiquitin proteasome system is a major component of the protein quality control system, and prevents the accumulation of defective proteins through the recognition, degradation, and removal of misfolded proteins.
When the amount of misfolded proteins produced exceeds the capacity of cellular protein quality control systems, in particular in neurons with the formation of protein aggregates, neurons undergo cell death, and misfolded proteins becomes a cause of neurodegenerative diseases (Parkinson’s disease, Alzheimer’s disease, Huntington’s disease). In addition, even if the amount of defective proteins produced is normal, a decline in the activity of protein quality control systems makes people susceptible to neurodegeneration.
While it is known that the ubqiquitin system and the proteasome play important roles in the clearance of misfolded proteins, it remains unknown how misfolded proteins are distinguished from native proteins, and how only the former are targeted for degradation. We aim to unravel the molecular mechanisms behind the degradation of cellular misfolded proteins.
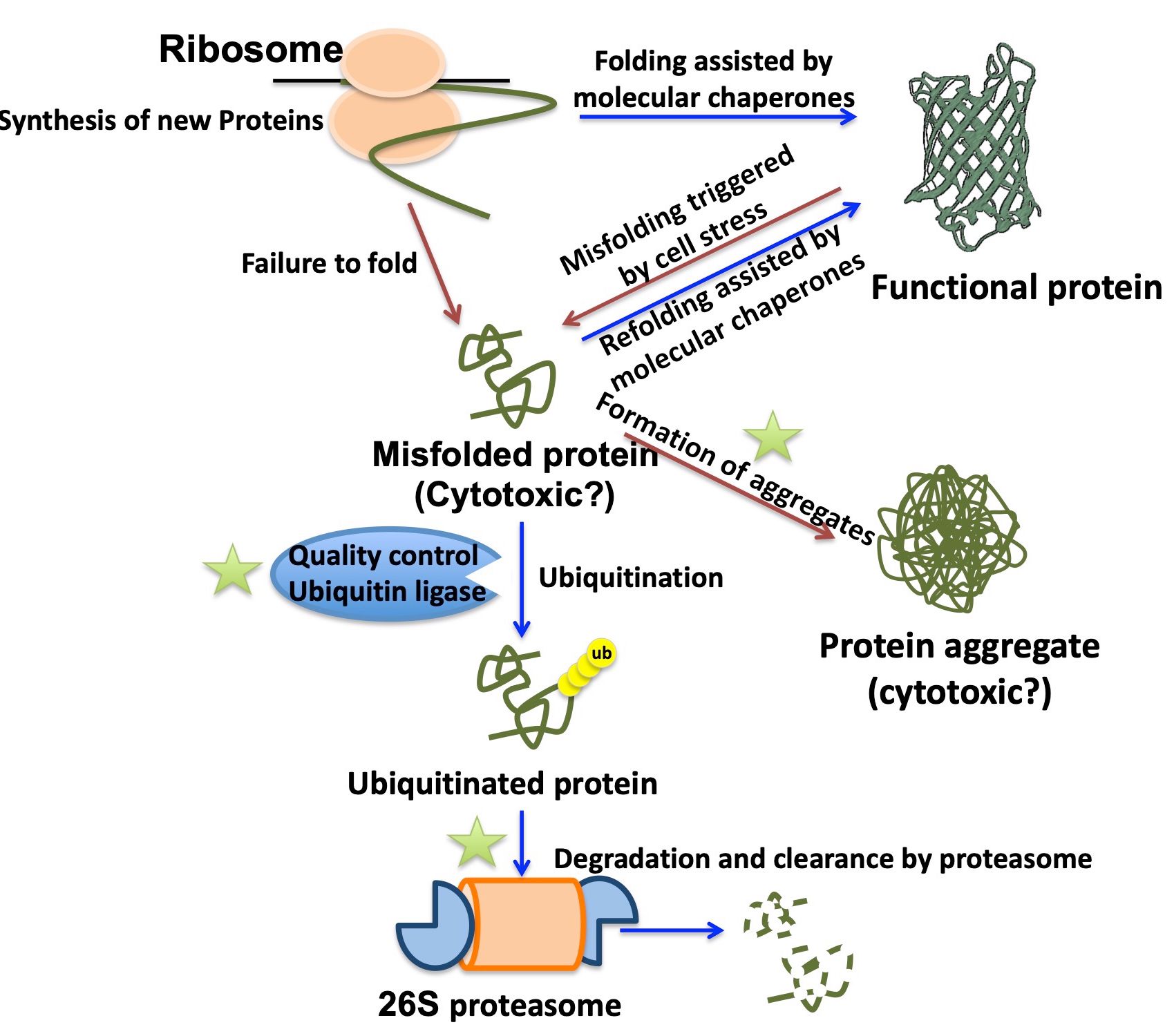
When the amount of misfolded proteins produced exceeds the capacity of cellular protein quality control systems, in particular in neurons with the formation of protein aggregates, neurons undergo cell death, and misfolded proteins becomes a cause of neurodegenerative diseases (Parkinson’s disease, Alzheimer’s disease, Huntington’s disease). In addition, even if the amount of defective proteins produced is normal, a decline in the activity of protein quality control systems makes people susceptible to neurodegeneration.
While it is known that the ubqiquitin system and the proteasome play important roles in the clearance of misfolded proteins, it remains unknown how misfolded proteins are distinguished from native proteins, and how only the former are targeted for degradation. We aim to unravel the molecular mechanisms behind the degradation of cellular misfolded proteins.